Targeting Immune Checkpoints as Cancer Therapy
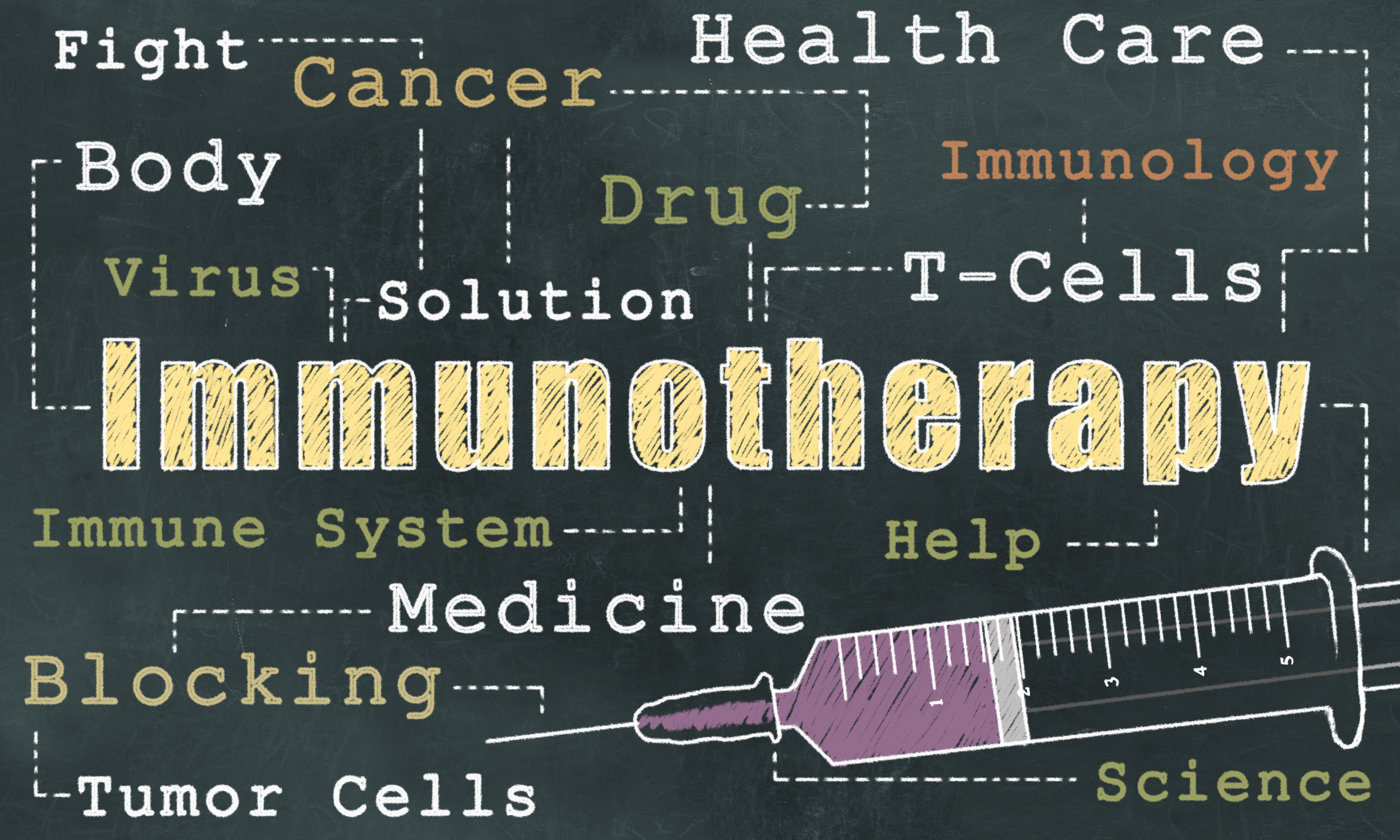
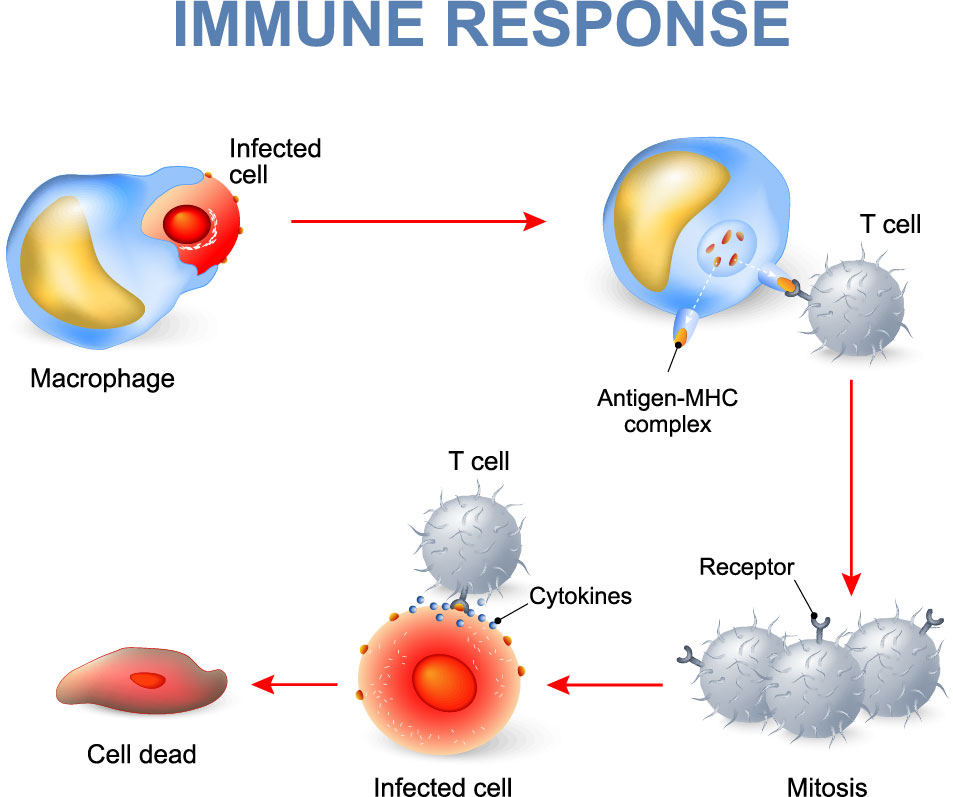
Figure 1. Illustration of T cell-mediated immune response upon signaling from an antigen presenting cell.
If signal 2 is co-inhibitory, controlled by B7 proteins binding to inhibitory immune checkpoint CTLA-4, then CD28 co-stimulation will be outcompeted and T cell activation will be suppressed (Fig 2). CTLA-4 and PD-1 further act by inhibiting TCR-induced cytokine secretion and proliferation, as well as glucose uptake and metabolism, resulting in T cell exhaustion3. This negative-immune regulation is needed to impede excessive immunity that, if left unchecked, could lead to damage to normal tissue or autoimmunity3.
Figure 2. Key ligand-receptor interactions between T cells and APCs. T cell activation follows after TCR recognition of MHC with co-stimulatory signal from B7-1 binding with CD28. T cells are deactivated if MHC-TCR recognition is followed by co-inhibitory signal from B7-1 binding with CTLA-4 or PD-L1 binding with PD-1.
However, we now know that cancer cells can express high levels of some immune checkpoint proteins, essentially exploiting T cell suppressive effects and evading the immune response for tumor survival3. As we learned earlier, antibodies can target immune checkpoint proteins and block binding at T cell-tumor cell interfaces (Fig 3), freeing T cells to attack tumor cells. Antibodies now designed to target checkpoint proteins, called immune checkpoint inhibitors (ICIs) (Fig 3), are the subject for numerous drug discovery and development enterprises and considered among the most rapidly emerging immunotherapies4.
Figure 3. Illustration showing checkpoint proteins PD-L1 expressed on tumor cells and PD-1 on T cells. Interaction between PD-L1 and PD-1 prevents T cells from destroying tumor cells (top, Before). Using immune checkpoint inhibitors to either PD-1 or PD-L1 allows the T cells to kill the tumor cells (bottom, After).
BioLegend is committed to empowering cancer research and advancements toward novel immunotherapies by providing trusted, expertly-crafted resources. Our portfolio includes Avi-tag biotinylated proteins, which offer consistent labeling, uniform orientation of the protein, equivalent bioactivity to that of non-biotinylated proteins, and lot-to-lot consistency. Learn about biofunctional recombinant proteins and functional antibodies we offer for immune checkpoints being evaluated as immuno-oncology targets, such as PD-1, PD-L1, and CTLA-4 that have transitioned from lab research to therapeutics. While successful, many patients show primary or acquired resistance to these particular ICIs, driving research toward additional ICIs, used either on their own or in combinatorial blockade with anti-PD-1/L1 and/or anti-CTLA-4 to synergistically enhance anti-tumor immunity5. For example, the B and T lymphocyte attenuator (BTLA, also known as CD272) is an immune checkpoint co-inhibitor expressed on numerous immune cells5. Also, CD80 and CD86, ligands that interact with CD28 to stimulate T cell survival, yield inhibitory signals and suppress T cell functions when they interact with CTLA-4. As a result, CD80 and CD86 are also considered candidate targets for therapy6. Approaches thus far described involve the strategy of blocking inhibitory immune checkpoint receptors. Another approach is to use agonists of co-stimulatory immune checkpoint receptors, such as CD40/CD40L, which are members of the tumor necrosis factor receptor superfamily (TNFRSF)7.
Researchers can also test strategies for stimulating anti-cancer immune responses with in vivo and ex vivo models using our bioactive GoInVivo™ antibodies. These antibodies are designed to target immune checkpoint markers, validated by functional assay, and competitively priced for bulk purchase.
Finally, the ability to accurately quantify expression levels of checkpoint molecules is critical to ongoing biomedical research with the goal of developing potential immunotherapeutic interventions. Learn about our LEGENDplex™ Human Immune Checkpoint Panel 1, a flow-based multiplex assay that allows for the simultaneous quantification of 12 key immune checkpoint biomarkers (sCD25 (IL-2Ra), 4-1BB, sCD27, B7.2 (CD86), Free Active TGF-β1, CTLA-4, PD-L1, PD-L2, PD-1, Tim-3, LAG-3, and Galectin-9). The panel has been validated for use on cell culture supernatant, serum, and plasma samples (Fig 4).
Figure 4. Serum and EDTA plasma samples were obtained from healthy donors and patients with confirmed breast and lung cancer. Serum and plasma samples were run neat and analytes analyzed using LEGENDplex Human Immune Checkpoint Panel 1 (12-plex).
The growing field of immunotherapy has revolutionized cancer treatment by boosting the body’s own immune system to target and eliminate malignant tumors8. Along with ICIs, bispecific antibodies and CAR-T cells are considered the most promising immunotherapies9. In this blog, we focused on immune checkpoints; cell surface molecules that regulate T cell functions and activities, but which can also be exploited by tumor cells to evade immune control. That discovery triggered intense research into ICIs that have now transitioned to the clinic and are considered standard treatment for a number of cancers. However, there are still unmet medical needs and our scientists are ready to support R&D that will lead to the next generation of innovative treatments.
References
- “Nobel Prizes 2018.” NobelPrize.org, www.nobelprize.org/prizes/medicine/2018/advanced-information/. Accessed 1 Feb. 2023.
- Kuzume, Ayumi et al. “Immune-Checkpoint Blockade Therapy in Lymphoma.” International journal of molecular sciences vol. 21,15 5456. 30 Jul. 2020, doi:10.3390/ijms21155456. PubMed.
- Baldanzi, Gianluca. “Immune Checkpoint Receptors Signaling in T Cells.” International journal of molecular sciences vol. 23,7 3529. 24 Mar. 2022, doi:10.3390/ijms23073529. PubMed.
- Djurian A, Makino T, Lim Y, Sengoku S, Kodama K. Dynamic Collaborations for the Development of Immune Checkpoint Blockade Agents. J Pers Med. 2021 May 24;11(6):460. doi: 10.3390/jpm11060460. PMID: 34073680; PMCID: PMC8225058. PubMed.
- Qin, Shuang et al. “Novel immune checkpoint targets: moving beyond PD-1 and CTLA-4.” Molecular cancer vol. 18,1 155. 6 Nov. 2019, doi:10.1186/s12943-019-1091-2. PubMed.
- Bolandi, Nadia et al. “The Positive and Negative Immunoregulatory Role of B7 Family: Promising Novel Targets in Gastric Cancer Treatment.” International journal of molecular sciences vol. 22,19 10719. 3 Oct. 2021, doi:10.3390/ijms221910719. PubMed.
- Tang, TingTing et al. “Molecular basis and therapeutic implications of CD40/CD40L immune checkpoint.” Pharmacology & therapeutics vol. 219 (2021): 107709. doi:10.1016/j.pharmthera.2020.107709. PubMed.
- Ribas, Antoni, and Jedd D Wolchok. “Cancer immunotherapy using checkpoint blockade.” Science (New York, N.Y.) vol. 359,6382 (2018): 1350-1355. doi:10.1126/science.aar4060. PubMed.
- Mukherjee, Anirban Goutam et al. “Role of Immune Cells and Receptors in Cancer Treatment: An Immunotherapeutic Approach.” Vaccines vol. 10,9 1493. 7 Sep. 2022, doi:10.3390/vaccines10091493. PubMed.
Follow Us